The Biological Approach to Behaviour: Neuroplasticity
Introduction to Neuroplasticity
Neuroplasticity is the brain's ability to change by modifying synaptic connections between neurons typically caused by genetic and environmental factors. Genetic factors refers to the normal pre-programmed development of the brain, while environmental factors include injury, brain damage or acquiring new skills. Naturally, there are different scales of neuroplasticity - from synaptic plasticity to cortical remapping.
Synaptic plasticity is the ability of the neuron to form new synaptic connections and break up the old ones. Synapses refer to the places where neurons connect and communicate with each other. Each neuron possesses anywhere between a few to thousands of synaptic connections. If two nearby neurons are frequently activated at the same time, a synaptic connection may gradually form. Similarly, if two neurons are rarely activated together, the existing connection may gradually fall apart. These connections can be with itself, surrounding neurons or even neurons in other regions of the brain. Connections between neurons can change in response to neural activity, making the brain flexible and adaptable.
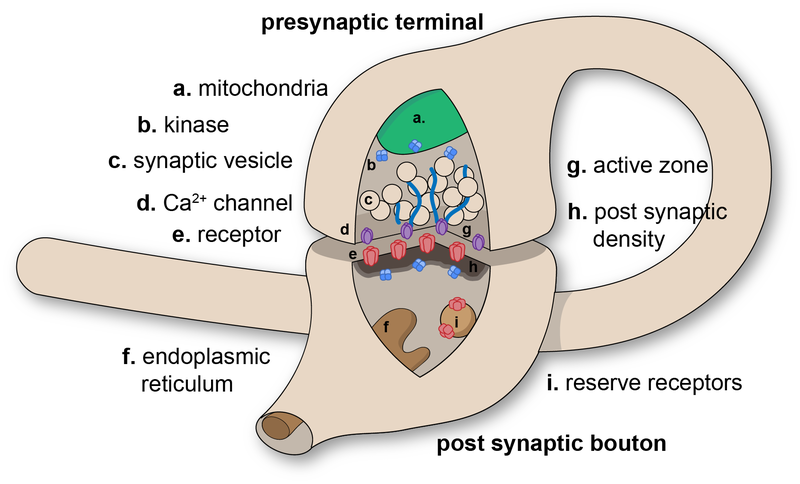
Cortical remapping is the phenomenon where one area of the brain assumes the functions of another brain area. One possible cause for this could be, for example, injury. Cortical maps are collections of minicolumns in the brain cortex that have been identified as performing a specific function. Cortical mapping is a procedure that involves the identification localization of functional areas within the cerebral cortex.
Remapping of the Sensory Cortex
One of the earliest studies of neuroplasticity was done by Merzenich et al (1984), on the level of cortical remapping. During this experiment, researchers studied the cortical representation of the hand in eight adult owl monkeys.
There were three steps to the procedure.
- First, sensory inputs from all the hand digits (fingers) were mapped in the cortex. This was done by inserting electrodes in the cortical area known to be responsible for sensation from the hand; researchers stimulated various areas on all the fingers individually and noted which electrode was responding to the stimulation.
- The second step was to amputate the third digit (the middle finger) on the monkey's hand.
- The final step was to do a remapping of the cortical area responsible for sensitivity from the hand and note how it had changed sixty-two days after the amputation.
Researchers found that there were five distinct areas in the brain, each responsible for one finger. Adjacent fingers were represented in adjacent areas in the cortex. It was found that the adjacent areas to digit 3, those responsible for sensitivity from digits 2 and 4, spread and occupied parts of the unused area after the amputation. In other words, the areas responsible for digits 2 and 4 became larger while the areas responsible for digits 1 and 5 stayed the same. Therefore, based on the results of the experiment, it was concluded that cortical remapping of sensory inputs from the hand occurs within 62 days in owl monkeys.
Mechanism of Learning - A Form of Neuroplasticity
Believe it or not, neuroplasticity occurs regularly in our daily lives as the mechanism of learning causes the brain to gradually reshape itself. To put it in plain terms, when you learn, your brain gradually reshapes itself.
Draganski et al (2004) conducted a study to find out whether the human brain structure can really change in response to environmental demands. To do this, the researchers randomly allocated a sample of volunteers into one of two groups: jugglers and non-jugglers. Precautions were made to ensure that both groups had no experience in juggling prior to the experiment. The first brain scan was performed at this point. Then, participants in the juggler-group spent three months learning a classic juggling routine with three balls. The second brain scan was performed. After that, the participants spent another three months where they were instructed not to practice juggling. The third brain scan was performed after the so-called "non-practice" period. The control group (the non-jugglers), on the other hand, lived their daily lives and had their brains scanned three times as the jugglers did.
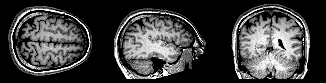
At the start of the experiment, a comparison of brain scans in the two groups showed no clear differences in brain structure. However, at the second scan, the juggler group had substantially more grey matter in some areas of the cortex, particularly in the mid-temporal area in both hemispheres. These areas are known to be associated with movement coordination. At the time of the third scan, after the no-practice period, the differences decreased but were still present to a certain extent. Researchers also noticed that there was a correlation between juggling performance and the amount of change. Participants who trained better exhibited brain changes that were more pronounced.
Based on the results shown from the experiment, it was concluded that learning a simple juggling routine can cause certain areas of your brain to grow. However, when you fail to practice, they shrink back significantly, although not to the initial state.
Another study, by Draganski et al (2006) was conducted on 38 medical students learning a large amount of abstract material in preparation for an examination in medicine. The first scan was acquired three months before the examination; the second scan one to two days after the examination; and the third scan three months after the examination. Results from the scans showed that there were no differences in regional grey matter at baseline, yet there were two major changes taking place in the brains of the medical students. Firstly, there was an increase of grey matter in the parietal cortex in both hemispheres which did not decrease by the time of the third scan. Therefore it can be concluded that studying for an examination in medicine has a more lasting impact on the brain than learning a juggling routine. Secondly, there was an increase of grey matter in the posterior hippocampus which gradually increased from the first scan to the third.
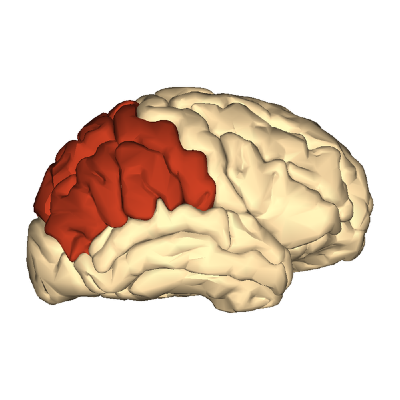
These observations have justified previous knowledge that these areas of the brain are involved in the formation of new memories. However, what surprised researchers was the fact that the changes in the posterior hippocampus continued to occur subsequent to the examination. Based on previously discovered properties of the hippocampus, the researchers suggested that learning increased grey matter in the posterior hippocampus but examination stress decreased it. (Stress is known to reduce grey matter volume in hippocampal regions.) Therefore, the negative influence of the examination stress was corrected and the lost hippocampal volume was restored after the examination.
Conclusions about Applications of Neuroplasticity
The aforementioned studies provide evidence to show how the brain is a plastic structure that can adapt itself to the demands of the environment. The question is, how can we use this for practical purposes? Can we rewire the visual cortex of blind people so that they can "see" using their other senses? Can we implant electrodes into the motor cortex of a person with a mechanical prosthetic limb and train the brain to control the artificial limb? The reality is, the potential applications of neuroplasticity are countless; especially when it comes to sense substitution which is when another sense is used to make up for a lost sense.
Paul Bach-y-Rita was one of the first neuroscientists to introduce this concept. Among one of his first inventions was a chair that allowed congenitally blind people to "see". It was a chair with a large camera behind it, with a purpose of scanning the are and converting the image to an electrical signal sent to 400 vibrating stimulators attached to the back of the chair. The blind subject would sit in the chair and learn to recognise visual stimuli from the vibrating signals against his or her back. This way subjects gradually learned to recognise images such as objects, shadows and faces. The brain would interpret tactile information and convert it into visual images, which requires neuroplasticity to change the visual cortex and other brain areas.
All in all, neuroplasticity allows the brain to constantly rewire and adapt, leading to structural and functional changes over time. This process is crucial for a range of human activities, including learning, memory formation, and overall development. If the brain lacked neuroplasticity, we wouldn't be able to acquire new skills, adapt to new experiences, or retain knowledge, making many of the defining aspects of human behavior and growth impossible.